People Listing
Cliff Burgess
Professor
Research Interests: Black Holes and Quantum Information, Dark Energy and Dark Matter, Early-Universe Cosmology, Effective Field Theories, Quantum Hall Systems and ADS/CFT Duality
Interesting times for particle physics
This is a very exciting time to be working on Particle Physics, since we sit at the threshold of new discoveries likely to be driven by experiments at the Large Hadron Collider.
My own wild oats were sown working in string theory, but my research interests have since taken a more phenomenological and cosmological turn. At present my interests lie at the interface between string theory and lower-energy physics, with a particular emphasis on how the discovery of branes (and the realization that we may be trapped on one) may have observable consequences in experiments and in cosmology. In particular, I am working on the implications I believe this may have for Dark Matter, Dark Energy, Inflation and for the Large Hadron Collider.
To the extent that there is a theme to my research, it would be the use of effective field theory techniques throughout high-energy physics and other fields. These techniques permit a general understand of the low-energy (or long-wavelength) behaviour of any physical system. They are particularly apt for our present situation in particle physics, where it appears that the energy scale of any unknown physics beyond the Standard Model is high compared to those that are experimentally accessible. But part of the beauty of these techniques is that they are also applicable in other areas of physics and so they permit a unified perspective towards theoretical physics as a whole.
Supervisor Letter:
Cliff Burgess
Department of Physics & Astronomy
McMaster University
Dear Prospective Graduate Student,
Hi there, Having a wonderful time – wish you were here!
I’m not quite sure what this kind of a letter should say, but if you are reading this you are probably thinking about graduate school and you might be wondering what it is like to do graduate studies in particle theory. So the opening line pretty much sums it up!
I am a theorist and my training is in high-energy physics, which in cartoon form is the search for the most elementary building blocks of Nature and the forces through which they interact. This search culminated about 30 years ago in the Standard Model of particle physics, which provides a really good description of all of the experiments which have been done to date (with the exception of recent observations of neutrino masses). The bad news is that this was all done back in the stone age (30 years ago), but the good news is that the Standard Model contains within itself an indication that it must fail at distances which are just below the shortest ones which can be reached by present-day experiments. This is one of the main motivations for building the Large Hadron Collider, a new accelerator facility at CERN in Geneva which hopes to find experimental evidence for what lies beyond the Standard Model.
Theorists like me are trying to identify what might be seen. An important clue in this regard comes from reconciling quantum mechanics with gravity, since this seems to point to a very particular kind of theoretical framework called string theory. In string theory the elementary building blocks turn out to be little one-dimensional objects having a length but no width. All known particles are regarded in this framework as modes of vibration of one of these strings. This picture turns out to be theoretically very restrictive, and much of my current research is aimed at seeing if this restrictiveness can be parlayed into an understanding of what might be seen once experiments begin to probe physics beyond the Standard Model.
There is lots to do, and if you are interested the best thing is to contact me (at cburgess@physics.mcmaster.ca) for more up-to-date information.
 
Soo Hyun Byun
Professor
Research Interests: Development of advanced radiation detectors for the nuclear industry and in space
My research group has been focusing on advanced radiation detector and nuclear instrumentation developments. Particularly, we developed the THick Gas Electron Multiplier (THGEM) detector, an advanced gaseous radiation detector, which showed an outstanding performance in contrast to the traditional gaseous detectors. We also developed new digital signal processing systems for radiation spectrometry and imaging. Selected current research projects are listed below.
THGEM neutron imaging detector development In 2013, we successfully developed a THGEM X-ray imaging detector.
The core work of this project was to devise a simple and efficient 2-D position readout board. For 2-D position encoding, we developed a digital signal processing system using time to digital converters and a field programmable gate array processor, which required an extensive amount of work in terms of circuit fabrication and processor programming. The detector was successfully tested for alpha and X-ray imaging. Founded on this work, we are currently designing an efficient neutron converter in order to develop a digital neutron imaging detector.
THGEM multi-element neutron dosemeter and 2-D neutron-gamma dosemeter developments
Tissue-equivalent gaseous proportional counters are made of tissue-equivalent plastics and filled with tissue-equivalent gases, which makes them the most accurate device for measuring neutron dose. However, their neutron detection efficiency is pretty low and therefore, they have not been employed for weak neutron field measurements typically encountered at nuclear power plants. To address this fundamental low efficiency problem, we have developed a “multi-element” detector consisting of a number of gaseous sensitive volumes using our THGEM technology. In order to optimize the multi-element design, we carried out extensive Monte Carlo simulations. With this Monte Carlo outcome, we accomplished a quantitative analysis for the neutron efficiency dependence on the multi-element geometry for the first time. We have built a prototype multi-element detector consisting of 7*3 gaseous volumes and its tests are currently underway.
Another advanced dosemeter we have been developing is a 2-D neutron-gamma dosemeter, which aims to measure a spatial distribution of the neutron and gamma-ray doses. The core work of this project was to build a multi-input digital signal processing system which can analyze signals from 25 detectors in parallel and real time. A preliminary result showed that the 2-D dosemeter shows the spatial neutron and gamma dose distributions quite accurately. Moreover, the digital processing system showed an excellent processing speed and energy resolution. Comprehensive tests for this 2-D detector are currently underway.
Low-level gamma-ray spectrometry
In ultra-low level gamma spectrometry, a common challenge is that owing to its weak activity, the counts from a sample are buried under background radiation counts. For the multi-photon emitters like 60Co and 26Al, important radionuclides of interests for meteorites and/or nuclear reactor samples, this challenge can be overcome by operating detectors in coincidence mode, in which case a large fraction of the background counts can be rejected while most cascade gamma-ray counts from a sample are saved. Although this principle has been well known, no systematic studies have been done in terms of optimizing the detector arrangement and segmentation to accomplish the best analytical performance.
This study will focus on measuring low level 26Al, 60Co and others radionuclides of interests. We will optimize the detector size and array pattern through an extensive Monte Carlo simulation study. The project scope also includes pulse processing development for multiple detectors in a digital architecture and investigation of optimizing time pickoff algorithm.
Alan Chen
Professor
Research Interests: Experimental nuclear astrophysics; radiative alpha- and proton-capture reactions; charged-particle transfer reactions; recoil separators and magnetic spectrographs
Pat Clancy
Assistant Professor
Research Interests: The study of novel materials using x–ray and neutron scattering techniques
Ryan Cloutier
Assistant Professor
Research Interests: exoplanets, planet formation, planet composition, M dwarfs, observational astronomy
Ryan Cloutier’s research focuses on the detection and characterization of the galaxy’s most common planets around its most common stars. These exoplanets have sizes between that of the Earth and Neptune and do not have an analog in our solar system that we can study up close. His interests are in using observational techniques to understand the compositions of these so-called super-Earths and sub-Neptunes, to inform our understanding of how these planets form and whether their surface conditions may be hospitable for life.
Robert Cockcroft
Associate Professor
Research Interests: Astronomy, Indigenous astronomy, teaching track
Kari Dalnoki-Veress
Professor
Research Interests: Soft and living matter at surfaces and interfaces: Polymeric materials, but also C. elegans (a small nematode), bacteria, living cells and soft-colloidal systems.
The main focus of our research group is the study soft and living matter at surfaces and interfaces. The physics of soft materials is distinct from hard matter as the weaker intermolecular bonds result in a large response to external stresses. We study mainly polymeric materials, but also C. elegans (a small nematode), bacteria, living cells and soft-colloidal systems. Understanding material properties at a fundamental level is crucial to the development of new technologies. A surprising aspect of these materials is that on small length scales, like in thin films or coatings, these materials can have properties that differ vastly from those of bulk systems. The differences can be the result of molecules being confined or because the interface plays a greater role in smaller systems. 
While retaining significant activity in fundamental studies of polymeric systems on the nano-scale, in recent years our research has also moved into new directions. These include greater efforts at the interface of biology and soft matter, as well as a bourgeoning emphasis on complex fluids beyond polymeric. Current directions of our research in soft and living matter can be summarized under four broad and overlapping areas: 1) Biophysics: micro-swimmers, collective motion, and vesicles. 2) Complex fluids at interfaces: elastocapillarity and soft colloids. 3) Fluids on the micro/nanoscale: nanorheology, confinement, the glass transition. 4) Self assembly and patterning.
The broad range of problems studied in the research team is largely the result of a somewhat opportunistic approach to research. Simply stated, we often stumble upon interesting detours that have resulted in exciting and fun research. Over time some diversions have developed into fully established research directions. Though the projects are diverse, they share a common theme of small length scales, surfaces, and interfaces, with a common physical foundation. The main experimental tools used focus on characterization of surfaces, imaging, and force measurement.
Supervisor Letter:
Dear Prospective Group Member,
We are always looking for new enthusiastic and ambitious researchers to join our team. For details please see our group web page as well as my letter to prospective members
If you are interested in working in our team, please contact me to discuss the possibilities and also feel free to contact any previous members of the group.
Kari
Kevin Diamond
Associate Professor
Research Interests: Radiation therapy & dosimetry, Medical Physicist at the Juravinski Cancer Centre
Cecile Fradin
Professor
Research Interests: Motions and conformational changes of proteins, pore formation in lipid membranes, swimming bacteria.
Experimental Molecular Biophysics
Our focus is the experimental investigation of the dynamics of single molecules inside biological systems using optical tools as well as x-ray and neutron scattering.
Dynamics is essential to the survival of the cell, which is a biological unit in permanent evolution, and which has to be able to process and react to information. Dynamical processes inside the cell happen on a very wide range of length and time-scales, and are governed by complex and intricate rules and mechanisms. At the scale of the molecule, they are of interest for the physicist as well as for the biologist, since they involve basic transformation of chemical or thermal energy into mechanical energy. In order to unravel their exact mechanisms, in vivo quantitative measurements at the single molecule level are required, which recent developments in the domain of fluorescence microscopy and single molecule detection now gives us the opportunity to do.
- Check our group website for detailed information on current research projects.
- For information on undergraduate studies in Biophysics check our Honours Biophysics website.
Supervisor Letter:
Dear prospective trainee,
students in my group are carried out experimental research at the frontier between physics and the life sciences. The main focus of our research is single molecule dynamics, that is the motions and conformational changes that biomolecules undergo while carrying out their function. It entails observing molecules both in isolation (single molecule work) and when part of a larger unit (the cell), using a range of different biophysical techniques.
Since I started at McMaster in 2001, I have supervised or co-supervised 2 post-doctoral fellows, 6 Ph.D students, 7 M.Sc. students and countless undergraduate students. Trainees in my group may have Physics, Chemistry, Life Sciences or Engineering degres, and in fact working as part of a team with different scientific background is one of the thing I enjoy most about my work.
As a research supervisor I am committed to the education and growth of every trainee in the group, whether your goal is a carrier in academia or just the opportunity to learn a little bit about Biophysics over the summer. I believe this can be achieved through research projects that are both relevant and well-defined, access to state-of-the-art equipment, close collaborations with researchers in the life sciences, regular meetings between supervisor (me) and trainee (you), a supportive group atmosphere, and the opportunity to attend summer schools and conferences.
Do not hesitate to contact me or anybody else in the group for more information.
Cecile.
Bruce Gaulin
Distinguished University Professor
Research Interests: Geometrically frustrated magnets, Quantum Magnets with singlet ground states, High temperature superconductors
I am an experimental condensed matter physicist, working in the general area of scattering studies of exotic ground states in new, mostly magnetic, materials. As described below, this means that we (my grad students, postdocs, collaborators and myself) make new materials which we think will have interesting and exotic ground states, and then take these materials to forefront neutron and x-ray scattering facilities in North America and around the world. We perform scattering experiments on these new materials and then work either independently or with our friends in theory to interpret the experiments, and thereby shed light on the exotic properties of the new materials.
How do we generate materials which exhibit exotic ground states? Well, we try to incorporate features into the crystal structure and the nature of the magnetic moments which encourage fluctuations, and thereby make it difficult for the material to find an ordered state at low temperatures. We have three features we can work with: we can make crystal architectures which are likely to show geometrical frustration; we can make magnetic crystals which have quantum magnetic moments in them, especially s=1/2 magnetic moments; and we can make three dimensional crystals which are made up of an assembly of low dimensional substructures, like stacks of quasi-two dimensional planes of atoms.
At present we have three themes to our work:
Geometrically frustrated magnets: these are magnetic materials which possess local geometries and magnetic interactions whose combination is incompatible with long range order. The easiest to appreciate occurrence of geometrical frustration happens with the combination of antiferromagnetism and triangular geometries. The tetrahedron is to three dimensions what the triangle is to two dimensions, so this is a common, but poorly understood occurance in three dimensional crystal structures made up of networks of interconnected tetrahedra.
We have been particularly active studying cubic “pyrochlore” magnets, which can be thought of in terms of magnetic moments decorating a network of corner sharing tetrahedra. Such materials have much difficult reaching a magnetically ordered state at low temperatures, and can display a host of exotic, disordered magnetic ground states such as spin liquid, spin glass, and spin ice states. We have devoted much effort to studying the rare earth titanate family of cubic pyrochores. You can look up our recent work on Yb2Ti2O7, Ho2Ti2O7, Er2Ti2O7, and Tb2Ti2O7 under publications. Grad students Jacob, Pat, Kate, and Katharina, and postdoc Jeremy have been leading these and related projects.
Quantum Magnets with singlet ground states: these are magnetic materials comprised of s=1/2 quantum magnetic moments decorating various lattices. One interesting and basic result of quantum mechanics is that while the classical picture of ferromagnetism corresponding to all spins in a solid pointing in the same direction is also valid when quantum mechanics is taken into account, the classical Neel state for antiferromagnetism is not correct when a fully quantum treatment of antiferromagnetism is necessary.
In these cases, which is really all cases as quantum mechanics applies to everything, antiferromagnetic interactions between the quantum moments can result in the formation of local singlets and an overall non-magnetic ground state. While the ground state is a non-magnetic singlet, the excited states, which we can probe by neutron spectroscopy, are triplet states and multi-triplet states. Application of a magnetic field Zeeman-splits the triplet state and a strong enough magnetic field can drive the energy of one of the triplet of excited states below the ground state. The net effect is that triplets can begin to condense into a “sea” of singlets and crystallize within the non-magnetic ground state in phenomena very closely related to Bose condensation.
We‚Äôve focused on two types of quantum antiferromagnets which display singlet non-magetic ground states: the quasi-two dimensional Shastry-Sutherland system SrCu2(BO3)2 and quasi-one dimensional spin-Peierls systems. In the first case, the s=1/2 magnetic moments are already arranged into pairs which then form singlets at low enough temperatures. In the spin-Peierls case, uniform chains of s=1/2 magnetic moments must spontaneously “dimerized” to form a necklace of pairs of ions which then form singlets. In the latter case, the quantum magnetism and the lattice on which the s=1/2 moments reside are strongly coupled. You can look up some of our recent publications for both these materials. The SrCu2(BO3)2 was led by Sara Haravifard, who just finished her PhD and is now a postdoc at the University of Chicago. The spin-Peierls work on TiOBr and TiOCl is being led by Pat Clancy.
High temperature superconductors: Actually high temperature superconductors are also quantum magnets – doped quantum magnets, decorating a low dimensional structure, a two dimensional one in this case. So these materials combine a couple of the general themes we are interested in.
Many scientists have been interested in these materials for more than 20 years, mostly because they display superconductivity at temperatures as high as ~ 150 K. We have focused on the magnetism in the so-called “parent” materials in the High Tc family, and on how this magnetism is modified at relatively low doping. The “doping” means that we start out with a pure “parent” compound, La2CuO4, and then replace a small percentage (like 1 -10 %) of the La3+ with Ba2+. Its known that the effect of this doping is to remove s=1/2 quantum magnetic moments from the CuO2 plane in these materials. The magnetic phase diagram of these materials in this low doping regime is remarkable (at least to me!), in that the magnetism evolves from a strong, three dimensional, commensurate antiferromagnet through a couple of different, but related two dimensional, incommensurate antiferromagnetic states, one of which also displays the superconductivity. Our work on La(2-x)Ba(x)CuO4 is being led by grad students Greg, Jerod and Kate.
Supervisor Letter:
Bruce D. Gaulin
Professor and Brockhouse Chair in the Physics of Materials
Director, Brockhouse Institute for Materials Research
Dear Prospective Graduate Student;
Thank you for your interest in experimental condensed matter physics at McMaster. I am primarily interested in studying exotic phases of matter by neutron and x-ray scattering techniques. These phases are typically generated by unusual magnets which remain in entropy-dominated, disordered states down to very low temperatures; by quantum magnets where a non-magnetic singlet ground state occurs at low temperatures, and by high temperature superconductors which may display novel “stripe” phases in which magnetism and superconductivity co-exist in an inhomogeneous fashion.
My graduate students and postdoctoral fellows study these exotic materials by carrying out neutron and x-ray scattering experiments to determine the atomic and magnetic structure and dynamics of the materials, typically as a function of temperature. I presently have six grad students, Jacob Ruff, Pat Clancy, Kate Ross, Katharina Fritsch, Greg van Gastel, and Jerod Wagman, and one postdoctoral fellow, Dr. Jeremy Carlo. Jacob and Kate came to my group from the University of Waterloo, Pat from St. Francis Xavier University, Katharina from University of Leipzig in Germany, Greg and Jerod from the University of Toronto and Jeremy recently completed his PhD at Columbia University in the USA.
I have supervised or co-supervised about 25 very talented grad students and postdocs as a faculty member at McMaster. They have all gone on to exciting careers as faculty members themselves (at the U. of Toronto, Edinburgh U., Kent State U., the Technical University of Munich), as scientists at prestige government laboratories (at the Staecie Institute of NRC, NIST, Oak Ridge National Laboratory, Los Alamos National Laboratory, Lawrence Livermore National Laboratory, Argonne National Laboratory, Helholtz Zentrum Berlin, Defence Research Establishment, Ottawa), and in high tech industry (at Seagate, JDS Uniphase, Read Rite Corp, and Moli Energy).
Neutron and x-ray scattering are very similar techniques, although they provide different and complementary information regarding the structure of materials. A student who has been trained in one of the techniques will have an easy time with the other, as they have so much in common with each other. I have built up and operate an x-ray scattering lab at McMaster, based on an 18 kW rotating anode x-ray generator, and we have the capability to carry out scattering studies over a range of temperatures from about 400 K to 0.3 K. We carry out our neutron scattering experiments at leading international facilities such as the Chalk River Laboratories in Canada, NIST, Oak Ridge, and Los Alamos in the USA, ISIS in the UK, the HZB in Germany, and the ILL in France.
A crucial first step in these studies is to produce the novel materials in pristine, single crystal form. McMaster has extensive facilities, the best in Canada and among the best in the world, for crystal growth and sample preparation. We have built up a new state-of-the-art floating zone optical image furnace laboratory, with two image furnaces, and these have been optimized for the growth of large single crystals of novel oxide materials, like frustrated pyrochlore magnets and high temperature superconductors.
McMaster has tremendous strengths in the study of new materials, and particularly exotic magnets, metals, and superconductors. I work closely with Professors Graeme Luke, Tom Timusk, Takashi Imai, and Maikel Rheinstadter in experimental physics, while it is a great advantage for us to have superb condensed matter theorists with related interests, as we do with Professors Berlinsky, Kallin, Sorensen, and Lee. All of us are part of the Canadian Institute for Advanced Research’s (CIfAR) Program on Quantum Materials, which gives us an opportunity to interact with other outstanding scientists from Canada and around the world on a regular basis.
Please visit my website for much more detail. I am always happy to chat with students about my research interests or physics in general. Please feel free to contact me with any questions you may have! You can also contact my grad students and postdocs directly, if you’d like to get an insider’s perspective on life as a Mac physics grad student. Their contact info is on my website, and they would also be happy to hear from you.
Sincerely yours;
Bruce Gaulin
Paul Higgs
Professor
Research Interests: Evolution of Bacterial Genomes and Horizontal Gene Transfer, The RNA World and the Origin of Life, Codon Usage, Translational Efficiency and Dynamics of Ribosomes
Biophysics and Computational Biology
I use computational and theoretical methods to study problems in Biophysics, Molecular Evolution and Origins Research. Details of my research interests are on my home page.
Supervisor Letter:
Dear Prospective Graduate Student,
I am currently looking for new graduate students to work on Evolution of Bacterial Genomes, the RNA World and the Origin of Life, and Codon Usage and Translational Efficiency. There is a link to each of these projects on my home page.
My work is interdisciplinary, and I like to have students from several different backgrounds. All projects will involve some mixture of the following:
¬?¬†¬†¬†¬†¬†¬†¬† Developing and testing mathematical and theoretical models
¬?¬†¬†¬†¬†¬†¬†¬† Interpretation of biological sequence data
¬?¬†¬†¬†¬†¬†¬†¬† Developing software and databases for computational biology
I am associated with several different graduate programs at McMaster. You should apply to whichever fits your background and future career goals the best. The links below should take you to the pages describing the admissions for the different programs.
Physics – This is my home department. I am looking for someone who is interested in developing mathematical models, theories and simulations to understand problems at the boundary of biology and statistical physics.
Biochemistry – I am also a member of this department and can I am looking for someone who is interested in molecular evolution or computational modeling of cellular processes.
Biology – I am an associate member of this department and I would be looking for a cosupervised student with a member of the biology department in the areas of evolution, population genetics and origins research.
Astrobiology – This is a new program run by members of the Origins Institute. Students should apply to one of the departmental programs (e.g. Physics, Biochemistry or Biology), and can additionally become part of the astrobiology program if their research topic is appropriate.
Computational Science and Engineering – This program would suit students who want a strong training in computational and numerical methods. I am looking for someone who wants to design and build bioinformatics software and databases for use in scientific research.
If you would like more information on any of these things, please contact me via the email above.
Paul Higgs.
Takashi Imai
Professor
Research Interests: Quantum spin liquids, low dimensional quantum magnetism, quantum criticality, unconventional superconductivity, strongly correlated electrons, NMR spectroscopy, low temperature physics.
Unraveling The Mystery of Quantum Materials
We conduct experimental research on a variety of topics in quantum condensed matter physics using NMR (Nuclear Magnetic Resonance) as the primary experimental probe.¬† NMR is a technique invented by physicists, but it is used for MRI in hospitals as well.¬† Nuclear spins interact with electrons in their vicinity via hyperfine interactions.¬† By observing how nuclear spins respond to perturbations caused by radio frequency pulses we apply, we figure out what electrons are doing.¬† In other words, we use the nuclear spins as a “spy” of electrons in quantum solids.¬†
We conduct NMR experiments using our own NMR spectrometers and superconducting magnets in our laboratory.  Our recent research topics include: quantum spin liquids on the Kagome-lattice Heisenberg antiferromagnet, quantum spin liquids on the Kitaev honeycomb lattice, quantum criticality of the transverse field Ising chain, and strongly correlated electros in copper-oxide high temperature superconductors. 
We are always looking for enthusiastic students, who want to participate in our research.  If you wish to know more details, please visit our homepage or contact me.
https://physics.mcmaster.ca/~imai/  
Research interests:
Quantum spin liquids, low dimensional quantum magnetism, quantum criticality, unconventional superconductivity, strongly correlated electrons, NMR spectroscopy, low temperature physics.
Supervisor Letter:
Takashi Imai
Department of Physics & Astronomy
McMaster University
We are a research group specialized in experimental investigation of quantum materials.¬† Our primary experimental probe is Nuclear Magnetic Resonance (NMR) conducted at cryogenic temperatures near absolute zero.¬† We investigate a variety of materials, such as the “spin liquid” on the kagome Heinsenberg model, iron-based high temperature superconductors, etc.¬† We try to focus our attention on the most important problems, and aim for publishing a small number of high-impact papers.¬† We are always looking for enthusiastic students who want to participate in the cutting edge research in our lab. ¬†¬†
Takashi Imai, Ph.D.
Hari Kunduri
Associate Professor
Research Interests: Mathematical and theoretical aspects of black holes, within the framework of general relativity and its extensions in string theory
Sung-Sik Lee
Professor
Research Interests: Strongly Correlated Quantum Many-Body Systems, Quantum Field Theory, Quantum Critical Phenomena, Non-Fermi Liquids, Holography
Condensed matter systems, that include insulators, semiconductors, metals and superconductors among many others, consist of many particles, of the order of ten to the twenty three. At the microscopic level, the dynamics of individual particles is governed by the well established theoretical framework, quantum mechanics. However, it is extremely difficult to understand and predict macroscopic behaviors of a whole system, starting from the microscopic theory. For the past 30 years, it has become clear that understanding macroscopic properties is not a matter of mere application of the known physical laws. Instead, collective behaviors are governed by novel organizing principles, which are not manifest from the microscopic rule of dynamics. Currently, the primary goal of my research is to identify new dynamical principles that are behind novel emergent phenomena in gapless phases of matter such as unconventional metals and systems near quantum critical points, and to apply those principles to understand physical properties observed in experiments. To achieve the goal, I use various theoretical tools, including quantum many-body theory and quantum field theory. I am also interested in the interdisciplinary research areas between condensed matter physics, string theory and gravitational theory. Recently I have been developing the notion of `quantum renormalization group’ where coupling constants are promoted to quantum mechanical operators to make a connection between general D-dimensional quantum many-body systems and (D+1)-dimensional quantum theory of gravity via holography. I am trying to use the quantum renormalization group scheme to understand strongly coupled field theories that are hard to solve otherwise.
 
Sung-Sik Lee @ Perimeter Institute
Supervisor Letter:
Dear Prospective Graduate Student,
My main research area is strongly correlated many-body system. When many particles (as many as ten to the twenty-third power of particles) are strongly correlated (interacting) with each other, they can behave very differently than either when there are only a few particles or when they are not strongly correlated. Because of the enormous amount of degrees of freedom and the strong correlation between them, it is impossible to predict measurable physical properties of a system from the dynamics of individual particles. However, as we observe them in a longer distance scale, we notice that there are some orders (patterns) in their fluctuations. Sometimes, these emerging patterns can be described in surprisingly simple and beautiful ways. The goals of my research are to understand various emergent phenomena in correlated quantum many-body systems using quantum field theory and holographic description.
If you want to know more about my research, please contact me via email.
Sung-Sik Lee
Graeme Luke
Professor
Research Interests: Muon spin relaxation, Crystal growth, Electronic properties, Magnetism, High pressure, High magnetic fields
Research in the Luke group focusses on experimental studies of so-called quantum materials which include exotic superconductors and novel magnetic systems. Systems which combine superconductivity with magnetism are of special interest.
At present, our group is actively studying a number of systems including pnictide and cuprate high temperature superconductors in addition to a variety of so-called heavy fermion systems where electron-electron interactions results in extremely large carrier effective masses. Some of these heavy fermion systems exhibit a number of exotic ordering phenomena ranging from magnetic order and superconductivity to so-called hidden order where a thermodynamic phase transition is apparent in a range of measurements, but the nature of the ordered state is unknown.
Supervisor Letter:
Graeme Luke
Department of Physics & Astronomy
McMaster University
Dear Prospective Graduate Student,
Thank you for your interest in condensed matter physics at McMaster. My research is in the area of highly correlated electron systems, especially exotic superconductivity and magnetism. At present I have one graduate student (on the border between MSc and PhD); a second student has just graduated. In the experimental correlated electron physics group as a whole (Gaulin, Luke, Timusk) there are generally 6 students, 2 or 3 postdocs and a few research scientists. Previous students in my group have gone on to work in industry, academia and teaching (in Thailand) and have presented their work at both national (Toronto, Quebec, Vancouver, Victoria) and international (Switzerland, Japan) conferences. I’m an associate in the Canadian Institute for Advanced Study (CIAR) Superconductivity program; each year this program has a summer school for graduate students as well as an annual meeting (for students and faculty).
Much of my research involves the technique of muon spin rotation (µSR), which roughly speaking, is a type of magnetic resonance (like NMR) performed using implanted muons. These experiments are performed mainly at TRIUMF in Vancouver, but also at ISIS (near Oxford, England). Click here for an introduction to the µSR technique.
My present interests include high temperature and other exotic types of superconductivity and low dimensional magnetism. Some of the systems I’m working on are:
- Sr2RuO4 is a 1.5K superconductor. As a result of the work of our group and others, Sr2RuO4 is thought to be a p-wave superconductor where the electrons forming the Cooper pairs are arranged with parallel spins.
- La2CuO4+x and La2-xSrxCuO4 are high Tc superconductors which can undergo both superconducting and magnetic/charge ordering (in the form of charge/magnetic stripes). We are using µSR to study the interplay of superconductivity and magnetism in these materials.
- There a a number of magnetic materials where either the effective dimensionality of the magnetic interactions or the presence of geometrical frustration prevents the formation of an ordered state. Various quantum ground states can subsequently emerge including Anderson’s resonating valence bond (RVB) singlet state, a spin Peierls state, a spin plaquette singlet and others. We are using ¬µSR to identify these states and to characterize their excitations.
In addition to my work in µSR, I have a laboratory at McMaster equipped for low temperature magnetic, electrical transport and thermal measurements. The two main systems in my lab are a Quantum Design SQUID magnetometer for measurements from 1.8K to 800K in magnetic fields up to 5.5T and an Oxford Instruments Maglab ExaSystem for ac-susceptibility, specific heat and electrical transport measurements in magnetic fields as high as 9T and temperatures down to 1.5K. Furthermore, we have excellent facilities for materials preparation including two floating zone optical image furnaces which allow us to grow large single crystals of extreme purity.
There are openings in my research group for one or two new graduate students, either at the MSc or PhD level. I have ongoing projects in high Tc and heavy fermion superconductivity as well as low dimensional and frustrated magnetism. Our world-class crystal growth facilities allow us to study virtually any system of interest. New projects of mutual interest are also possible. Please send me an email if you’d like to discuss possibilities for graduate work in my group.
Graeme Luke
Fiona McNeill
Professor
Research Interests: Development of radiation-based biomedical devices for the painless, in vivo measurement of toxic metals such as Pb, As, F, Al and Gd in bone, liver, and kidney.
Trace Toxic Elements
The main focus of my research is the development of biomedical devices based on radiation physics techniques for the in vivo measurement of trace toxic elements. In the last few years, my graduate students have built the first in vivo systems in the world for the painless, non-invasive and low dose measurement of arsenic, gadolinium and fluoride. We use two main techniques for these devices; neutron activation analysis (NAA) and x-ray fluorescence (XRF) analysis.
In addition to building biomedical systems, I apply them to studies of human health and exposure. My research has helped the understanding of human health effects from lead (Pb) exposure; my work has shown that Pb can result in elevated blood pressure and early menopause in women. Recently we discovered that people living in Hamilton have measurable levels of fluoride in their bones and a major source of exposure is tea drinking. We have been studying gadolinium and this element is used in image enhancement drugs for MRI. The Gd may be detaching from the chelate which could be potentially toxic, so we are developing systems to measure long term uptake of detached Gd in bone.
Some of the reasons I study toxic metals are explained in the Research2Reality video which can be found at http://research2reality.com/video-categories/health/ under ‘measuring health effects of metal exposure’.
Radiation Tools in Cultural Heritage
In recent years, I have used radiation tools in the study of art and cultural heritage. I was a member of a team who studied nine works of art from the McMaster Museum of Art. This resulted in an exhibition, The Unvarnished Truth: Exploring the Material History of Paintings, which is travelling across Canada until 2017. The work is also presented in the interactive website http://theunvarnishedtruth.mcmaster.ca/ .
Low Dose Radiation Effects
For the last few years, I have been collaborating with Professors Colin Seymour and Carmel Mothersill from the Department of Biology. We have been studying the effects of low level exposure to radiation types that include x- and Œ?-rays and neutrons. We have recently shown that cells, when exposed to radiation, emit a UV signal which can cause effects in nearby cells that were not exposed to the initial radiation. The UV causes a ‚Äòhalo‚Äô of effect. We are continuing to explore whether this UV signaling is an important aspect of low dose radiation effects.
Reza Nejat
Associate Professor
Research Interests: Introductory physics, teaching track
Duncan O'Dell
Professor
Research Interests: Ultracold atoms
Ultra-cold atoms
One of the best ways to understand a complex system is to study its fundamental components. To better understand the behaviour of atoms, they can be chilled to a millionth of a degree above absolute zero. “Ultra-cold atoms are the coldest things in the universe that we know about, probably the coldest things that we will ever make,” said Duncan O’Dell, assistant professor of atomic, molecular and optical physics in the Department of Physics and Astronomy at McMaster University.
The study of ultra-cold atoms began in 1995 with the development of the first Bose-Einstein condensate, in which gaseous bosons are cooled down to almost absolute zero. The discovery received the 2001 Nobel Prize in Physics. “They were able to cool a bunch of atoms so that they all wound up in their quantum mechanical ground state, which is the lowest energy you can get anything,” O’Dell explained. This relatively new field of research also encompasses more traditional areas, such as condensed matter physics, nuclear physics and quantum optics.
At temperatures approaching absolute zero, ultra-cold atoms are almost motionless, except for some residual movement called zero point energy. In addition to their reduced movement, ultra-cold atoms behave more like waves than billiard balls. Quantum fluids like Bose-Einstein condensates are unlike typical fluids like water because they remain in a liquid state even at extremely low temperatures. Their constant movement due to their zero point energy prevents the liquid from freezing into a solid. “What they represent is our greatest control over nature,” said O’Dell. “When you can control nature at the single atom level, but also do it with an arbitrary number of atoms, you’re really king.”
Ultra-cold atoms enable atomic clocks to make very precise measurements of time. They are also used in interferometers, which measure interference between two beams of light or matter. As the light beams travel along different paths, they experience slightly different environments, which affects the phase of the light. When the beams recombine, the interferometer measures the phase difference as a product of interference.
Matter wave interferometers use waves of atoms directed along paths using electromagnetic fields. They can be used to measure gravity across very short distances. Since the atoms’ wavelength is much smaller than that of light, atom interferometers are much more sensitive than light interferometers. “You could have atoms go on two different trajectories: one close to a heavy block of material and one a bit further away and you look at the differences in their behaviour,” O’Dell explained.
For professors like O’Dell, the learning process never stops. The department’s positive learning environment fosters knowledge sharing between faculty members, even though their research interests are incredibly diverse. “Physics is a unified whole,” said O’Dell. “Although we’re all working on different subsystems, there is a lot of overlap between the general ideas. My colleagues are certainly experts, so I know there’s a huge reservoir of information I can draw upon.”
Supervisor Letter:
Dear Prospective Graduate Student,
I am currently looking for one student who would like to do their MSc/PhD at McMaster in the theory of ultra-cold atoms, beginning September 2010 or thereabouts. My research focuses on the quantum dynamics of simple systems, and ultra-cold atoms provide an excellent setting for this. I currently have two graduate students: Prasanna Balasubramanian, and Nick Miladinovic. Prasanna is studying ultra-cold atoms inside optical cavities which is a project that combines quantum optics with cold atom physics and has applications in the precision measurement of gravity (the least well understood fundamental force). Nick is studying methods for transferring light between two or more optical cavities, which is a project that combines quantum optics with adiabatic quantum mechanics and has applications in quantum information science. Both these projects are in collaboration with the experimental group run by Professor Ed Hinds at the Centre for Cold Matter. I also have research projects in collaboration with other theory groups in the general area of Bose-Einstein condensates.
Here are some reasons why I am currently working on ultra-cold atoms:
- They are simple and controllable systems that strike at the heart of quantum mechanics. For example, the creation of Bose-Einstein condensates in atomic gases (2001 Nobel prize in Physics for Cornell, Ketterle, and Wieman) created a macroscopic quantum system that can be easily probed and manipulated.
- Theory and experiment work hand in hand in this field. Beware: your theory might well be tested in the laboratory before you’ve finished your PhD!
- They combine physics from two major disciplines: Atomic, Molecular and Optical physics (which includes quantum optics), and Condensed Matter Physics. The field is therefore rich in opportunity and benefits greatly from cross- fertilization. As a student in this area you can gain experience in a broad range of subjects.
- Ultra-cold atoms are being used to address both fundamental questions, e.g. quantum phase transitions, or superfluidity, as well as technologically relevant questions such as the possibility of using ultra-cold atoms to realize the quantum logic gates necessary to build a quantum computer.
If you would like to know more about ultra-cold atoms take a look at the popular article by James Anglin and Wolfgang Ketterle:
http://cua.mit.edu/ketterle_group/Projects_2002/Pubs_02/angl02_nature_insight.pdf
If you think you might like to do research in this area I encourage you to email me at dodell@mcmaster.ca .
Duncan O’Dell
Laura Parker
Professor
Research Interests: Observational studies of galaxy evolution, galaxy groups and clusters, and dark matter halos.
My research interests lie in the general area of observational cosmology and galaxy evolution. I am most interested in the processes of galaxy and structure formation and I work on problems related to the relationship between observed, luminous galaxies and the dark matter halos that host them.
Some specific problems we’re currently working on include: the dynamics and dynamical evolution of galaxy group systems, the dark matter halos of galaxies in different environments, the growth of central galaxies in groups and clusters, how group galaxy properties depend on the X-ray properties of the host group.
Prospective students? please read my letter to prospective graduate students, and feel free to contact me.
Supervisor Letter:
Dear Prospective Graduate Student,
I am an observational astronomer interested in how galaxies form and evolve. My group uses imaging and spectroscopic data from ground- and space-based observatories at many wavelengths to measure the properties of galaxies. We are particularly interested in the role that environment plays in galaxy evolution and so we study large populations of galaxies living in dense regions like galaxy groups and clusters. Using large samples of galaxies, we are trying to understand the physical mechanisms driving galaxy transformations over cosmic time.
Students in the group work on a diversity of projects ranging from constraining the amount of cold, star-forming gas that is stripped from galaxies when the fall into a cluster to comparing observed galaxies to those in state-of-the-art cosmological simulations.
In recent years much of our work has focussed on the local universe, where there are large sample of galaxies with multi-wavelength observations available, but with new facilities in the coming years we intend to extend our studies to more distant systems that we see when universe was a much younger and more active place.
I’m actively looking for enthusiastic students interested in galaxy evolution and observational cosmology. Please contact me at lparker@mcmaster.ca if you think this is a research area you might be interested in!
Laura Parker
Ralph Pudritz
Professor
Research Interests: Star formation, planet formation, astrobiology
I am a theoretical astrophysicist and my research focuses on star and planet formation. I completed my undergraduate studies at UBC in mathematics and physics. I then moved to the University of Toronto for my M. Sc. (in theoretical physics). I returned to UBC to do my Ph.D. in astrophysics under the supervision of Greg Fahlman, completing it in 1980. I took up an NSERC Postdoctoral Fellowship at the Institute of Astronomy in Cambridge (England). I went on to further postdoctoral research with Chris McKee and Jon Arons at the Astronomy Dept. at Berkeley, and with Colin Norman at the Johns Hopkins University. I joined the faculty at McMaster in 1986. Research Leaves and Fellowships over the subsequent years have taken me to many outstanding research centres including the Observatoire de Grenoble (1988, 1992), the Max-Planck Inst. for Astronomy in Heidelberg (1993), the Harvard-Smithsonian Center for Astrophysics (1993), the Max-Planck Institute for Astrophysics in Munich (1997), the Canadian Institute for Theoretical Astrophysics (CITA) in Toronto (1990 and 1997), Caltech (2001), and the Kavli Institute for Theoretical Physics (KITP) in Santa Barbara (2007/08).
I have been involved in many aspects of Canadian as well as international astronomy and astrophysics, having served on Time Allocation Committees (CFHT and JCMT), NRC Science Advisory Committees (Gemini, JWST), Visiting Committees (U.S. NRAO), Advisory Boards (HIA, CITA Council), and review committees. I chaired Canada’s decadal survey of Astronomy and Astrophysics – the NRC-NSERC Long Range Planning Panel (1998/2000) – and was the principal author of the LRP report; “The Origins of Structure in the Universe”. The LRP is playing the central role in guiding the development of Canadian astronomy in this decade and beyond, having involved Canada in ALMA, JWST, TMT, SKA, and several other important space and ground based telescopes and observatories.
Most recently, I spear-headed and am the founding (2004) Director of McMaster’s Origins Institute (OI). Its scientific mission is to engage in fundamental transdisciplinary research on the origin of structure and life in the cosmos. The scientific themes of the OI cover 6 broad themes in science: the origin of space and time (cosmology, early universe), structure in the universe (planets, stars and galaxies), the elements, life (astrobiology), species and biodiversity, and humanity. In addition to its research foci, the OI has developed a novel OI Undergraduate Research specialization. The OI is committed to public outreach and education through its award winning OI Public Lecture series and played an important role in the creation of the McMaster 3D theatre. The OI has also run major international annual scientific conferences on some of the most important questions in contemporary science.
Supervisor Letter:
Ralph Pudritz
Department of Physics & Astronomy
McMaster University
Dear Prospective Research Students:
My research focuses on the theoretical and computational study of star and planet formation, and astrophysical and planetary aspects of the origin of life including experimental work in our Origins of Life Lab. Star formation impacts a huge range of astophysics – from planet formation to galaxy formation and evolution and cosmology. Stars and planets form in protostellar disks and there are very deep connections between these subjects through them. The discovery of over 4000 exoplanets is driving a major revolution in astrophysics as we try to develop new theoretical models that can explain the wealth of new data and planetary populations ‚Äì such as the dominant SuperEarths. The characterization of the composition of the atmospheres of rocky exoplanets is one of the main drivers for our search for life in the universe. Members of my group perform a wide range of state of the art, high performance computing simulations of star formation ‚Äì from galaxy scales down to individual stars forming in their protostellar disks; planet formation and the properties of exosolar planets and their atmospheres; and work on early Earth and prebiotic physics and chemistry that lead to the origins of RNA. We connect our work with exciting observations from new observatories such as the James Webb Space Telescope, as well as the ALMA millimeter observatory https://www.eso.org/public/teles-instr/alma/ allow us to connect the work with new observations. I am also the Co-Investigator for our Origins of Life Laboratory in McMaster’s Origins Institute https://origins.mcmaster.ca/research/origins-of-life-laboratory/
There are many exciting research opportunities in my group. In addition to individual meetings, I have regular group meetings every week in which everyone discusses their results and ideas. Students and postdocs at all levels are well connected to one another as well as with the many external collaborators across the world, that we work with.
Research topics in my group:
Star Formation explores a wide range of interconnected problems starting from the scale of the formation of molecular clouds in galaxies, down to filamentary structure of molecular clouds, to the formation of star clusters within them, to the collapse of individual gas “cores” within such clustered environments (to form single or binary stars), and on down to the physics of protostellar disks through which gas accretes onto their central stars and from which highly collimated jets are launched. Much of the research involves state of the art 3D numerical simulations, most recently using the RAMSES Adaptive Mesh Refinement code. We are currently using RAMSES for multiscale galaxy simulations that allow us to trace star formation in magnetized galaxies all the way from cloud formation on many kpc scales, through to star clusters and over the next year or two ‚Äì down to the 100 AU scales needed to study massive star formation in clusters. See recent articles:
-Star formation in filamentary molecular clouds and a new paradigm for star formation: see our review Andr?® et al, (2014): https://arxiv.org/pdf/1312.6232.pdf
– The formation of massive stars: Klassen, Pudritz et al (2016):
https://arxiv.org/pdf/1603.07345.pdf
– The formation of star clusters: Howard, Pudritz, & Harris ( 2018):
https://arxiv.org/ftp/arxiv/papers/1808/1808.07080.pdf
-Theory and simulations of protostellar jets and outflows: see review Pudritz and Ray (2019):
https://arxiv.org/pdf/1912.05605.pdf
Planet Formation: is arguably one of the hottest topics in astrophysics, and indeed science, today. In my group we are investigating all aspects of planet formation, connection how planets form in protostellar disks, to their final orbits and chemical compositions. This “end-to-end” approach can be used to predict the composition of exoplanet atmospheres, which will be observed for the first time with JWST. This research involves theoretical work, as well as extensive use of astrochemistry codes and population synthesis simulations. Some recent papers in my group include:
- • A theory for planet traps and the migration of forming planets in disks (2011): https://arxiv.org/pdf/1105.4015.pdf
• Linking the composition of atmospheres to planet formation (2016): https://arxiv.org/pdf/1605.09407.pdf
• The combined effects of disk winds and turbulence for planet formation and composition (2022): https://arxiv.org/pdf/2207.01626.pdf
• Bifurcation of planet formation histories (2022): https://arxiv.org/pdf/2111.01798.pdf
Origins of life: focuses on the connection between protostellar disks, and the properties of pre-biotic chemistry on newly formed habitable planets. Nucleobases, amino acids, and fatty acids are found in meteorites and can be synthesized in 100 km parent bodies – planetesimals – which are also the building blocks of terrestrial planets. We have done calculations to show how these molecules are synthesized within planetesimals. Recent work focuses on understanding how meteoritic delivered biomolecules evolve on early planetary conditions, to result in the formation of RNA polymers – the first genetic materials. See our recent article:
• Fate of nucleobases in warm little ponds, upon delivery by meteorites to the Early Earth, see our Cozzarrelli prize paper; (2017): https://arxiv.org/pdf/1710.00434.pdf
• HCN synthesis in early Earth atmospheres leading to biomolecule formation (2022): https://arxiv.org/pdf/2201.00829.pdf
I have many interesting research projects within this broad set of themes. I will be happy to discuss these with you. If you are interested, please send me e-mail at pudritz@physics.mcmaster.ca or consult my departmental home page. I look forward to hearing from you!
With best wishes,
Ralph Pudritz
Fereshteh Rajabi
Assistant Professor
Research Interests: Data-oriented theoretical astrophysics, radio transients, masers, quantum optics, quantum simulation, astrophysics, laser physics, quantum optics, and ultracold atoms
Maikel Rheinstadter
Professor
Research Interests: Structure and dynamics of membranes and proteins using X-ray and neutron scattering techniques.
Proteins act like gatekeepers: they control what enters and leaves the cell. “They make sure that good things are transferred through the membrane to feed the cell and bad things stay out,” Rheinstadter explained. “We hope to use this knowledge to enhance or diminish the function of certain proteins.”
Proteins can be a cell’s best friend or worst enemy. Some proteins help defend the cell against disease while others can create holes in the cell membrane, causing the cell to die. Proteins are the first victims of infectious diseases. By understanding how proteins function at the molecular level, scientists can better understand how cells are damaged or die as a result of infectious diseases, which can lead to the development of more effective drugs.
After completing his PhD in condensed matter physics, Rheinstadter became interested in biophysics because he “wanted to do something more relevant,” but as he flipped through the pages of a biophysics textbook, his interest turned to disappointment.
“Everything had been done and discovered,” he said. ¬†Looking at the diagrams, he thought, “No one has seen this happen. These mechanisms are so small and the movements are so fast that we can‚Äôt look at them with a microscope or magnifying glass. Hardly anything has been seen, measured or quantified. This is what I thought I could do: come up with new, more powerful techniques to observe these fast motions.” He hopes these techniques will someday be used to develop better drugs.
Rheinstadter joined McMaster University in 2009 after completing research and academic appointments in Germany, France and the United States. “It’s a very good school with very positive energy in the department,” he said. “The people are outstanding, very friendly and helpful.”
Supervisor Letter:
Dear Prospective Graduate Student,
One of the major challenges of modern physics is how to contribute to biology and life-sciences including the emerging biotechnology and biomedical device industries, functional foods and nutraceuticals, but also the development of new biomaterials and pharmaceutical developments. ¬†There is a huge potential yet to unleash. ¬†In our Laboratory for Membrane and Protein Dynamics, we use X-ray and neutron scattering techniques to study molecular structure and dynamics in synthetic and biological tissue. ¬†We investigate for instance interaction of common drugs with biological tissue of different composition mimicking brain-like or muscle-like tissue.¬† We also study more fundamental aspects such as motion of lipids and proteins in membranes and formation and properties of nanodomains, so-called rafts.¬† Current projects include developing molecular models for the low-dose aspirin therapy and functioning of common drug enhancers.¬† Our laboratory is equipped with a biophysical preparation facility and operates BLADE (Biological Large Angle Diffraction Experiment), Canada’s most powerful in-house X-ray diffractometer dedicated to membrane research.¬† From the high resolution X-ray diffraction experiments we determine the molecular structure of biological tissue:¬† (1) the out-of-plane structure of the membrane to determine the precise location of the different molecules in the membrane with sub-nanometer resolution and (2) the lateral organization of the different molecular components in the plane of the membrane. You are welcome to explore our¬†research web page¬†if you want to find out more about our research.
As a student in my group you will be trained to work in a biophysical preparation suite and use unique in-house scattering instruments, such as a BLADE.  We also use scattering infrastructure at large scale facilities, such as the Canadian Neutron Beam Centre (CNBC) at Chalk River, the Spallation Neutron Source (SNS) in the US, ISIS in the UK and the ILL in France.  You will develop excellent organization and teamwork skills to prepare and conduct experiments at these facilities and interact with outstanding scientists.  Experiments using the in-house equipment will give you the time to develop your experimental skills.
You will learn to work in a team, however, take the lead in your own research project.  The outcome of your work will be published in high impact research journals.  This is a very important part of your training and will set the foundation for your future career.  You will learn to combine your creativity, your intellect, and hard work to accomplish your discoveries.
You will also have the opportunity to present your work at national and international conferences and workshops such as the Biophysical Meeting and the American Physical Society’s March Meetings, and more specialized neutron and membrane conferences. ¬†These experiences will not only build the reputation of the group as a whole, but also your own reputation and training as a young scientist.
If you are interested in joining our team, please contact me at rheinstadter@mcmaster.ca to discuss the possibilities. I will be more than happy to tell you more about potential projects.
I look forward to hearing from you,
Maikel Rheinstadter
Chris Rowley
Assistant Professor
Research Interests: Magnetic Resonance Imaging (MRI) and medical physics
Miranda Schmidt
Assistant Professor
Research Interests: Biophysics, teaching track
An-Chang Shi
Professor
Research Interests: Theoretical soft condensed matter physcis, Self-assembly of block copolymers, Statistical mechanics of macromolecules and bilayer membranes
I am interested in theoretical soft condensed matter physics, which is the study of materials such as polymers, liquid crystals, surfactant solutions (micellar solutions and microemulsions), colloidal suspensions (ink, milk, foams, and emulsions), and fluids. The most intriguing property of these materials is their ability to self-assemble into complex organized structures from nanoscopic to macroscopic length scales. Typical examples are micelles (finite aggregates of amphiphiles) and block copolymer microstructures. The past 20 years has seen steady growth in understanding of the physical properties of these complex macromolecular materials. Nonetheless, exciting and challenging problems remain.
In the past years my research has been focused on the statistical mechanics of structures and phase transitions in systems containing polymers, surfactants, and colloids. Examples of my studies include the phases and phase transitions of block copolymer systems, theory of inhomogeneous polymer blends, theory of polyelectrolyte solutions, dynamics of reactive polymeric systems, and theory of polymerization kinetics.
Brief Biography:
- 1982 B.Sc. Physics, Fudan University, China
- 1988 Ph.D. Physics, University of Illinois at Urbana-Champaign, USA
- 1988 Post-Doctoral Fellow/Research Associate, McMaster University, Canada
- 1992 Member of Research Staff, Xerox Research Centre of Canada
- 1999 Associate Professor, McMaster University, Canada
- 2004 Professor, McMaster University, Canada
Supervisor Letter:
Dear Prospective Graduate Student,
My research is in the area of soft condensed matter physics, which is the study of materials such as polymers, liquid crystals, surfactant solutions, colloidal suspensions, and biomaterials. The vast territory of these soft materials extends to plastics, pharmaceuticals, foodstuffs, textiles, proteins, and blood. One of the most intriguing properties of these materials is their ability to self-assemble into complex organized structures. The self-assembly of periodic ordered structures has become the basis for developing new materials and devices, such as photonic band-gap materials, nanoporous membranes, nanowires and high-density information storage. The prediction, design and control of ordered or partially-ordered structures on the nanometer scale has become a central focus of the materials community and is an essential ingredient in the quest for ever more useful and inexpensive devices. Theorists support this endeavor by proposing new types of self-assembled structures and by developing methods of rational design. Furthermore, the self-assembly of soft materials provides a challenging fundamental problem in statistical mechanics.
Recently thermodynamic properties of block copolymer systems have become a paradigm for the study of self-assembly. Block copolymers are macromolecules composed of chemically different blocks tethered together, which spontaneously form a variety of ordered phases with domain sizes in nanometer range (1-100nm). Understanding the structures and phase transitions in block copolymers has been one of the most active research areas in polymer science in the past two decades. My research addresses two key questions, why certain ordered structures appear and how these structures behavior, using a variety of analytical and numerical techniques.
My research group consists of three to four graduate students.  All the members in the group are involved in research topics in theoretical soft condensed matter physics. Collaboration within the group is strongly encouraged, although attention will be paid to the necessity of each student and post-doctoral fellow having their own core research problems. Please contact me for current graduate studies opportunities.
An-Chang Shi
Sergey Sibiryakov
Associate Professor
Research Interests: Nature of dark matter and dark energy, origin and evolution of structures in the universe, formulation of the quantum theory of gravity
Alison Sills
Professor & Chair
Research Interests: Blue Straggler Stars, Globular Cluster Formation and Dynamics
In my research, I use knowledge of stars and stellar evolution to understand unusual stars in dense places, their interactions and their effect on their environment.
A selection of questions that my group is interested in:
- Blue Straggler Stars
- What are the expected pulsation characteristics of collisional & mass transfer blue straggler stars? Are those in agreement with the observations?
- What happens to the angular momentum in a stellar collision and during the subsequent evolution of the product? Is this the same or different for binary mass transfer products?
- Globular Cluster Formation and Dynamics:
- How can we explain the multiple populations now seen in globular clusters? Are the current explainations viable? What other ingredients are required to fully understand this problem?
- What are the connections between local clustered star formation and the formation & evolution of globular clusters?
I answer these questions using a variety of theoretical techniques and computer codes. Depending on the situation, I use stellar evolution codes, smoothed particle hydrodynamic codes or stellar dynamics codes, running on computers which range from laptops to supercomputers.
I am an active member of collaborations with researchers from Canada, the US and Europe, including the MODEST (MOdelling DEnse STellar Systems) collaboration and the MYStIX collaboration.
Supervisor Letter:
Alison Sills
Department of Physics & Astronomy
McMaster University
Dear Prospective Graduate Student,
My work is theoretical, and covers stellar evolution, stellar dynamics, and hydrodynamics. I am interested in stellar populations that occur in unusual circumstances, such as stellar collisions in globular clusters. I study both the populations themselves and the information that the populations can give us about the environment they are in. While most of my work involves computer simulations, I also have experience working with optical observational data, particularly from the Hubble Space Telescope.
I have been at McMaster since July 2001. My most recent students worked on observations of globular cluster properties in M87 (with Bill Harris); theoretical models of star cluster formation (with James Wadsley); models of very young star clusters including both the stars and the gas; and on models of globular clusters that were formed in a dwarf galaxy which was then accreted by the Milky Way. I usually take on one or two summer or senior thesis undergraduate students as well. I may have room in my group for an exceptional graduate student this year, and have a variety of projects available, including some involving special-purpose computing hardware for stellar dynamics, binary star modelling, cluster formation and early evolution, and global cluster properties.
Astronomical research is a collaborative process, and I try to treat graduate students as collaborators as much as possible. The first few years of graduate school are an important transition from being an undergraduate student (when the answers to all questions are known) to becoming an independent researcher (when asking the right questions is as important a skill as finding the answers). All my students choose projects based on a combination of their interests and mine. MSc projects are better-defined from the beginning, and tend to involve more input from me, while PhD projects are more often suggested and planned by the students themselves. I encourage students to attend conferences, both in Canada and abroad, and I expect them to publish the results of their research in international journals at all stages.
Please contact me at  asills@mcmaster.caif you think this is a research area you might be interested in or if you have any other questions.
Alison
Erik Sorensen
Professor
Research Interests: Condensed Matter Theory. Computational Quantum Many-Body Physics, Frustrated Magnets, Quantum Information, Numerical Methods, Parallel Computing.
I’m originally from Denmark where I did my undergraduate work as well as a masters at the University of Aarhus.¬† I got my Ph. D. at the University of California, Santa Cruz, 1992, in Physics.¬† After my graduation from UCSC I spent two years (1992-94) as a postdoc at the University of British Columbia in the physics department.¬† From British Columbia I moved to Bloomington for a second postdoc (1994-96) at The University of Indiana Bloomington in the condensed matter theory group.¬† From December 1996 till the fall of 2001 I was working as a professeur at the Universit?© Paul Sabatier in Toulouse. The web-server (for the theory group) can be accessed here. The theory group is part of the larger institute IRSAMC which you can contact here. In the fall of 2001 I joined the department of physics and astronomy at McMaster University here in Hamilton, Canada. The web-site for the department is accessible here.
My research is within condensed matter theory, in particular within what is sometimes called strongly correlated systems, but I have also an interest in statistical mechanics. Here’s a recent movie made by Peter Hitchcock of bosonic currents appearing on a 2+1 dimensional space-time lattice during a Monte Carlo simulation.
Supervisor Letter:
Dear Prospective Graduate Student,
My research is centered in condensed matter theory and computational physics. In general I have in the last few years been working within the area of strongly correlated systems, with an emphasis on understanding the critical properties of such systems. I have worked on a number of problems related to low-dimensional magnetism in particular one-dimensional S=1 and S=1/2 quantum spin chains, investigating the different phases and excitations. I am quite interested in quantum phase transitions; notably I have been working on the transitions occurring in the quantum Hall effect and transitions in low-dimensional magnets. I have also done some work on superconductivity, looking at the superconductor to insulator transition. Recently, I have become interested in quantum entanglement and quantum information and the relations these concepts have to strongly correlated systems. Another recent topic is the experimental realization of optical lattices that have been used for trapping cold atoms. I have a strong interest in computational physics and numerical methods in general (as well as in analytical techniques) and a considerable part of my research tends to use what one might call advanced numerical techniques such as densitry matrix renormalization group methods and quantum Monte Carlo. Some of these methods are well suited for parallel computers such as the SHARCnet facility that we will be using.
My most recent Ph D students were Fei Lin, now pursuing a postdoc at University of Illinois Urbana Champaign, and Fabien Alet, who recently got a CNRS position in France. Fei’s Ph D work concerned strong correlation effects in fullerene molecules. Using large scale quantum Monte Carlo simulations he managed to determine the spectral functions for a single C60 molecule. Using this information, combined with cluster perturbation theory, one can gain significant insight into the physics of the molecular solids that C60 molecules form. These molecular solids are known to become superconducting at surprisingly high temperatures and Fei managed to clarify many aspects of a proposed purely electronic mechanism for the superconducting transition. Fabien Alet, used continuous time quantum Monte Carlo methods to study low dimensional magnetism and quantum impurities. Some of his results on low temperature susceptibility and on-site magnetization can be directly measured in NMR experiments. However, most of his thesis work was spent studying and developing efficient methods for doing quantum Monte Carlo simulations using worm algorithms. He succesfully developed a new Monte Carlo algorithm that we subsequently applied to study quantum phase transitions in bosonic systems with an unprecedented detail. My most recent M. Sc student was Peter Hitchcock, who moved to Cornell university for his Ph D. Peter did his Masters thesis on the persistent current in small mesoscopic rings with an impurity in them. He studied this problem using an almost exact Hirsch-Fye quantum Monte Carlo algorithm. For samples of recent papers I have written with students and postdocs, please see my web-page under “Recent Publications”.
Advanced numerical methods are crucial for the study of many modern problems in physics and is extensively used in R&D and in other areas of industry making use of C++ / F90. In my opinion, graduate students in the area of computational physics should have excellent job possibilities.
Erik Sorensen
James Wadsley
Professor
Research Interests: Computational Astrophysics: Cosmology, Galaxy Formation, Star Formation, Protoplanetary Disks, Hydrodynamics, Numerical Methods, Parallel Computing
I was born in Australia and I have been in North America (primarily Canada) since 1992, when I started Graduate work in Astronomy at the University of Toronto. Working with my supervisor Dick Bond at CITA I applied gasdynamical simulations to the Lyman alpha forest problem. I was a postdoc at the University of Washington working with Craig Hogan and the “N-body Shop” group where I wrote the Gasoline code with Joachim Stadel and Tom Quinn. I then came to McMaster to work with Ralph Pudtriz and Hugh Couchman in Physics and Astronomy. In 2002 I switched from full-time research to start spending time all over campus assisting researchers to develop parallel programs for the new SHARCNET supercomputer clusters. I was still involved in cutting edge astrophysics research and in July 2003 I took up a tenure track position with the Physics and Astromomy Department at McMaster.
Supervisor Letter:
James Wadsley
Department of Physics & Astronomy
McMaster University
Dear Prospective Graduate Student,
Computational astrophysics enables the astronomer’s dream of seeing stars and galaxies come to life and evolve before their eyes. Young stars can have substantial amounts of gas in disks around them. With computer simulations we have demonstrated that these disks spontaneously fragment under ideal conditions to create giant gas planets larger than Jupiter. We can also help the process by smashing disks together, shredding them into a string of brown dwarfs. We are also modeling entire clusters of young stars for a much more realistic and violent star and planet forming environment.¬† Gas fragments when it cools and radiation is how heat is lost.¬† We have developed numerical radiative transfer models to follow infrared radiation that dominates heating and cooling within dusty disks and star forming regions as well as UV radiation coming from massive stars and black hole accretion disks.
A major success of our recent cosmological simulations is the formation of realistic spiral galaxies on a supercomputer. The simulated disk galaxies form over billions of years, consuming gas and smaller galaxies in what is regarded as fairly quiet evolution and look remarkably like the Milky Way. With the resolution we can achieve on supercomputers, we have shown that small galaxies are sufficiently violent to gravitationally eject dark matter from their cores — solving a key mystery in cold dark matter. Dramatic merger events can transform quiet galaxies into the full range of galaxies types seen in the universe and also drive some of their gas and stars into voracious black holes at their centres. To model these galaxies even better we are developing detailed prescriptions for the gas and how it cools, black holes, how stars form and how stars die in energetic supernova explosions.¬† A key new development is the importance of clustered supernovae: hundreds going off in a small region.
My work involves computer simulations of astrophysical systems including gravity, gas, radiation and other physics. We have used the parallel Gasoline code to simulate planets, brown dwarfs, young stars, supermassive black holes, galaxies, galaxy clusters and large scale structure in the universe. Some recent papers are listed at NASA ADS. Images and movies can be seen at https://www.physics.mcmaster.
I currently have graduate students working on cosmological galaxy formation, protostellar disks, star formation and numerical methods. I like to have a hands-on, collaborative working relationship with students where we jointly contribute to projects. My students can be involved in picking the astrophysical questions to ask, astrophysical theory, developing parallel code, trying out new physical models in simulations, designing and running astrophysical simulations and/or matching results to astronomical observations according to their preferences and how they like to work with others in the group. Students also write papers and present results at conferences in Canada and internationally. I have collaborators in Zurich and Seattle and there are prospects for joint projects and exchanges with those universities. I have co-supervised several students and I am open to joint thesis projects with other faculty in the department. I also usually take on one or two summer, co-op or undergraduate thesis students every year.
I am interested in applications for graduate work at the M.Sc. or Ph.D. level in the areas listed above. I particularly encourage applications from students with both physics and programming skills.
I encourage you to email me: wadsley@mcmaster.ca or arrange to visit McMaster in person.
Regards,
James Wadsley
Doug Welch
Professor
Research Interests: Light Echoes from luminous transient events like supernovae
Doug Welch’s first glimpse of Saturn through a low-tech telescope when he was eight years old was all it took to inspire a lifelong fascination with astronomy. He is now a professor in the Department of Physics and Astronomy at McMaster University, where he studies the faint reflected light of exploding stars, known as supernova light echoes, and other cosmological phenomena.
“A supernova is one of the most influential events in the universe,” said Welch. “The vast majority of elements more massive than helium are created by massive star supernovae. These elements make up much of our own bodies. Supernovae change how quickly new stars can form, and they can actually trigger the formation of new stars when they blow up.”¬†
When certain stars die, they go out with a bang. There are two ways in which supernovae occur: one is a massive star that can no longer produce energy through nuclear fusion and collapses; the other is a white dwarf, which siphons off mass from an orbiting companion star. When the mass of a white dwarf reaches a certain level, it becomes unstable and blows up, producing a very distinct mix of elements.
In a given galaxy, supernovae are rare occurrences (only six have been observed in the past 2,000 years in the part of our galaxy which is visible from the position of the sun), but they can provide a wealth of information about the universe. Although hundreds of years have passed since the last supernova visible to the unaided eye exploded in our galaxy in 1604, astronomers can still study supernova light echoes, which are produced by light from the outbursts that are scattered toward Earth by interstellar dust. The longer path taken by the scattered light allows astronomers to study these centuries-old supernova outbursts with modern instruments.
“The supernova light echoes were the result of a dark matter search where we found a source of noise that we initially couldn’t understand,” Welch explained. “That ‘noise’ ended up being light echoes from ancient supernovae.”
Welch shares his interest in astronomy through public outreach. He coordinated the purchase of new projectors for the William J. McCallion Planetarium, which hosts public astronomy shows for children and adults. He is also part of the Slacker Astronomy podcast available on iTunes (www.slackerastronomy.org).
The department is “very much a community of like-minded people,” said Welch. “The thing that makes academic jobs fantastically better than most other jobs is that you’re always bumping into new people, new ideas and you’re always learning.” He credits his undergraduate and graduate students with keeping him on his toes. “You’re always being challenged in a way that never gets old.”
Supervisor Letter:
Dear Prospective Graduate Student,
I am not in a position to recruit additional graduate students for the academic year beginning September 1, 2024. Nonetheless, should you be accepted at that time and your primary supervisor sees value in my knowledge and expertise, I may be available to serve on your supervisory committee.
Since you have read this far, I will take this opportunity to introduce myself more completely! I am an observational astronomer who studies supernovae light echoes and variable stars. I have also been a member of the Science Team of the MACHO Project and SuperMACHO Project whose goals have been to determine the fraction of dark matter in massive, compact objects. The SuperMACHO Project discovered light echoes around centuries-old supernova remnants in the Large Magellanic Cloud – a finding that has made it possible to link remnants with supernova outburst light classifications. Since that time, members of my group and I have been working in collaboration with Armin Rest (STScI) to locate and study supernova light echoes from historical supernovae in the Milky Way and to study pre-supernova candidates such as eta Carinae.
My first two Ph.D. students were Phil Fischer and Patrick Cote. Phil obtained an NSERC PDF which he took at AT&T Bell Labs with Tony Tyson. Subsequently, he was awarded a NASA Hubble Postdoctoral Fellowship which he took at the Department of Astronomy at the University of Michigan at Ann Arbor. He worked as a research associate at CITA thereafter and was then employed by ScotiaCapital in the Trade Floor Risk Management Department. After defending his Ph.D. thesis, Pat became a Research Associate at the National Research Council’s Dominion Astrophysical Observatory in Victoria, BC. He, too, was offered a NASA Hubble Postdoctoral Fellowship but turned it down in favor of a Sherman Fairchild Prize Fellowship at Caltech. He then became a tenure-track Assistant Professor at Rutgers. In July 2004, he moved to the Dominion Astrophysical Observatory of the National Research Council’s Herzberg Institute of Astrophysics where he is now a Senior Research Officer.
My most recently graduated Ph.D. student was Brendan Sinnott whose Ph.D. thesis topic was determining the degree of asymmetry in the supernova SN 1987A from light echo spectroscopy.
In the last few years, I have been working on machine learning techniques to detect light echoes from large, wide-field survey facilities, including MegaCam on the Canada-France-Hawaii Telescope, DECam on NOIRLab’s Blanco 4-meter telescope at Cerro Tololo in Chile, and the Dragonfly Telephoto Array – a special-purpose facility located at New Mexico Skies which has the best low surface brightness sensitivity in the world.
Best regards,
Doug
Marcin Wierzbicki
Associate Professor
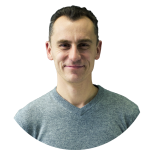
Marcin Wierzbicki
Associate Professor
Christine Wilson
Distinguished University Professor
Research Interests: Star formation and the interstellar medium in galaxies; observational radio and far-infrared astronomy
.
A bird’s eye view of the universe
Telescopes have come a long way since William Herschel invented the first reflecting telescope in 1789. The latest adaptation of his telescope for space astronomy, launched in 2009, has the largest mirror of its kind with a diameter of 3.5 metres and operates at infrared wavelengths of 60 to 600 microns.
“It takes pictures and measures spectra of planets in our solar system like Neptune and Jupiter as well as distant galaxies and everything in between,” said Christine Wilson, professor in the Department of Physics and Astronomy at McMaster University. “Herschel is good at seeing dust emission from galaxies and emission lines in spectra from gas in galaxies.”
Wilson has used the telescope to survey 13 nearby galaxies. “We use high resolution images to see what’s going on with those galaxies and interpret what’s happening in galaxies that are much farther away,” said Wilson. The Herschel mission ran for about four years. When the telescope ran out of liquid helium, a cooling agent, it stoppped working.
Wilson is part of a team that built SPIRE, one of Herschel’s three instruments. SPIRE collected data in the form of images and spectra. Atoms and molecules can be identified by the frequency they emit while the intensity of the lines they produce indicates their quantity. “Carbon monoxide emits very characteristic lines,” Wilson explained. “It’s a very regular pattern that’s easy to recognize.”
Initial spectral data from two galaxies showed strong lines, but the type of molecule producing some of the strongest lines was a mystery. “We didn‚Äôt know what it was,” said Wilson. “We didn‚Äôt know if it was this molecule or that molecule. If it was methanol, for example, we would have seen more lines.” After consulting with colleagues and the spectral line catalogue, the team concluded that they were looking at water.
“It was a bit of a eureka moment,” said Wilson. “The galaxy is the remnant of a merger between two galaxies. Two nuclei that came together can still be seen separately. It looked like water was coming from one nucleus and not the other. Why does one nucleus have water and the other doesn‚Äôt?” Although water is expected to be found in clouds, it’s usually absent when it freezes to dust particles and vapourizes when the dust is heated or shocked.
Wilson is currently focusing more of her research efforts on data from ALMA, the world’s most sophisticated ground-based radio telescope located¬† in the Chilean Andes. “ALMA can see really fine detail of star formation and galaxies,” said Wilson. Astronomers from around the world, including Canada, the United States, Europe, Japan, Taiwan, Korea, and Chile, use ALMA for a wide variety of research.
Research Interests
My work involves all aspects of observational star formation and the molecular interstellar medium, both in our own Galaxy and in other galaxies. I am particularly interested in the properties of giant molecular clouds, the nature of the interstellar medium in dwarf galaxies, the mechanisms regulating star formation rates and efficiencies in galaxies, and the properties of low-mass protostars in nearby molecular clouds. A large part of my observational work is concentrated in the regime of millimeter-wave radio interferometry, where high-resolution images of the emission from molecules in the interstellar medium can be obtained. However, many of these problems require a multi-wavelenght approach and so I have used a variety of optical and radio telescopes, including the Submillimeter Array (SMA), the James Clerk Maxwell Telescope (JCMT), and the Herschel Space Observatory . In 2013-2014 I was on research leave at the Atacama Large Millimeter Array (ALMA) and the North American ALMA Science Center .
Here are some of the projects that my group is currently working on:
- JINGLE, a survey of ~200 nearby galaxies in CO and dust emission using the JCMT
- a large survey of HI-selected galaxies within 25 Mpc to study the dense molecular gas associated with star formation, the gas-to-dust mass ratio, and to look for variations and correlations with galaxy type, mass, star formation rate, metallicity, etc. (The JCMT Nearby Galaxy Legacy Survey, with former graduate student Angus Mok, and roughly 50 collaborators).
- a detailed study of the dense gas in the nearest interacting galaxy system known as “the Antennae” using ALMA and Herschel (involving graduate student Ashley Bemis and former graduate student Max Schirm)
- a major survey of 14 nearby luminous infrared galaxies to determine the distribution, kinematics, and physical conditions of the gas and dust, to compare with simulations of galaxy mergers to understand how the gas and star formation rates evolve during the merger process, and as a well-studied local sample to understand high-redshift submillimeter galaxies (with former graduate student Kaz Sliwa and various collaborators)
From 1999-2014 I was the Canadian Project Scientist for the Atacama Large Millimeter Array (ALMA), which was recommended as the highest priority for Canadian participation in a major, new, ground-based observatory in the report of the Long Range Planning Panel, “The Origins of Structure in the Universe” . ALMA began Early Science observations in September 2011. I am an Associate Scientist with the SPIRE instrument for the Herschel Space Observatory , which operated from 2009 to 2013. I am also a member of the Board of Trustess of Associate Universities, Inc.
Here is where you can find my 2013-2014 sabbatical blog which has both science and personal entries.
 
Supervisor Letter:
Christine Wilson
Department of Physics & Astronomy
McMaster University
Dear Prospective Graduate Student,
Star formation is one of the critical processes that drives galaxy evolution in the local universe. Yet the underlying physics of the dependence of the star formation process on the properties of the interstellar medium, which provides the fuel for star formation, remains poorly understood. The structure of the interstellar medium itself can in turn be affected by the presence of star formation, through heating, ionization, and shocks, leading to a complex interplay and exchange of energy and matter between gas and stars.
My work involves all aspects of observational star formation and the molecular interstellar medium, both in our own Galaxy and in other galaxies. My recent work with my students and postdoc includes: multi-wavelength studies of gas and star formation in nearby galaxies (with Ryan Chown, currently a postdoc at Western University); a survey of the effects of environment on the molecular gas in galaxies (with Angus Mok, currently teaching at OCADU); a large survey of nearby luminous infrared galaxies to understand star formation in extreme environments (with Kaz Sliwa, currently employed by Miovision); a survey of the warm dense molecular gas in two nearby interacting galaxy systems  to determine the gas mass and and star formation properties (with Max Schirm, currently employed by Miovision); studying the critical phase in the formation of a massive star when its ionizing radiation starts to present a barrier to continued accretion (with Pam Klaassen, currently on staff at the Astronomy Technology Centre in Edinburgh), and measuring the mass function of massive clumps of gas in several nearby molecular clouds to try to understand the earliest phases of massive star formation (with Mike Reid, currently an Assistant Professor at the University of Toronto).
I am the only radio astronomy faculty member at McMaster and I also have experience working with data at optical and infrared wavelengths. I have been involved with the Atacama Large Millimeter Array (ALMA) for over a decade. ALMA is a large international collaboration involving Europe, North America, and Japan and recently completed its¬† construction in northern Chile. ALMA began “Early Science” observations in 2011 and my students and I have been successful in getting observing time with ALMA . In addition to observing with ground-based telescopes like the James Clerk Maxwell Telescope (JCMT) and the Submillimeter Array (SMA), I also use data from¬† the Herschel Space Observatory, which operated from 2009-2013. I’m currently putting a major effort into two new large surveys of nearby galaxies with the JCMT, as well as continuing involvement in large surveys using data from Herschel. All these surveys have lots of scope for graduate students to get involved.
I currently have 3 Ph.D. students (Nathan Brunetti, Hao He, Blake Ledger) and 2 M.Sc. students (Taavishi Jindel, Jennifer Laing) working with me, along with co-supervising Ph.D. student Nick de Marchi with Prof. Laura Parker. I plan to take on at most one new student in fall 2022; co-supervision with another McMaster faculty member is a possibility. I like to start new M.Sc. students on a project for which I have the data already. I have lots of possibilities for M.Sc. projects at the moment, such as studying the dust content of nearby galaxies to measure how the gas content varies with galaxy type or using Herschel observations to study the earliest phases of low mass or massive star formation in a nearby molecular cloud. With Ph.D. students, I like to give them time to define their own project and I don’t worry too much if it takes a year or so to settle on a project and start taking and analyzing data. My Ph.D. students often do a lot of observing and rapidly reach the point where they are doing all their own observations. There is a lot of scope for potential Ph.D. projects in the surveys I’m involved with, or you can define your own project from scratch.
Please feel free to contact me if you think this is a research area you might be interested in or if you have any other questions.
Sincerely,
Christine Wilson